Physical failure of a prop blade or hub in flight is far more likely to kill you than an engine failure. So why do many pilots ignore the relatively infrequent maintenance needed by their constant-speed propeller? Read more →
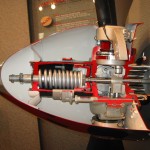
Physical failure of a prop blade or hub in flight is far more likely to kill you than an engine failure. So why do many pilots ignore the relatively infrequent maintenance needed by their constant-speed propeller? Read more →
Tires are probably the least sexy part of an aircraft: a ball of hot, stinky, greasy rubber. it’s also the airframe component which absorbs the most punishment, so they deserve a little attention every now and then. Read more →
Part two of the aviation myths series continues as I examine misconceptions about maintenance, shock-cooling, 40-hour minimums, stalls, and tailwheels. Read more →
My three-part series on aviation myths starts with: logging IMC, flying without charts, perfect eyesight, TBO, and owner-produced parts. Read more →